Carbon and Water Footprints of Diet Choices
Individual diet change has been promoted by many animal advocates and environmentalists. Acknowledging that social movements are far from being homogenous, we believe that identifying points in common across social movements is a first step to build connections between individuals making efforts to create a better world. In particular, promoting individual diet change seems to be a clear point in common between the animal advocacy movement and the environmental movement, as many organizations and individuals from both communities agree that our dietary patterns should move away from products sourced from animals.
Many animal advocates promote individual diet change as a way to help animals, while many environmentalists recommend it as a strategy to tackle environmental problems. We have already examined the impacts of individual dietary choices on the lives of farmed animals in effects of diet choices, and here we will examine the impacts of dietary choices from an environmental perspective.
We recognize that environmental problems deserve our attention, not only because they affect the survival and well-being of present and future human beings, but also because they may threaten the survival and well-being of many other animals.1 The relation between animal welfare and environmental resources is a topic worth investigating, but that is not our purpose here. Instead, we aim to engage with the environmentalist movement by examining research studies that assess the environmental impacts of dietary choices.
The environmentalist community has developed a large body of research to assess the environmental impacts of food production and consumption. Animal agriculture has come into focus as one of the human activities with highest environmental burdens, especially since the publication of Livestock’s Long Shadow by the Food and Agriculture Organization (FAO) in 2006.2 This comprehensive report suggested that the environmental impacts associated with the animal agriculture sector were much larger than commonly thought. It highlights not only their significant impacts on climate change, but also their contribution to other serious environmental issues such as land degradation, water pollution, and loss of biodiversity.
An increasing number of studies have evaluated the potential impacts of dietary change in tackling environmental problems by using different methodological approaches. Quantitative methods such as calculating the ecological, carbon, and water footprints have become increasingly popular for assessing food products and diets in terms of their impacts on different environmental aspects. As a first approximation to the multiple environmental impacts of dietary choices, we will examine the carbon and water footprints of food products and various dietary patterns in order to compare animal-based and plant-based diets in terms of their impacts on climate change and water scarcity. By selecting these impacts, we are not denying the importance of examining other significant environmental impacts, such as biodiversity loss and land use and degradation.
The extent to which environmental problems harm animals is a matter of some debate. We do not intend to enter that debate here; we will focus instead on recognizing the environmental impacts of our dietary choices.
Steinfeld, H., Gerber, P., Wassenaar, T., Castel, V., Rosales, M., & de Haan, C. (2006). Livestock’s Long Shadow: Environmental Issues and Options. Rome: FAO.
Table of Contents
Carbon Footprint of Diet Choices
Since the pre-industrial era, increasing emissions of greenhouse gases (GHGs) generated by human activities have accentuated Earth’s greenhouse effect.1 This interference in the global climate system is commonly known as “climate change,” which not only involves rising global temperatures, but also melting ice caps and glaciers, rising sea levels, ocean acidification, and extreme weather events (IPCC, 2014). The impacts of climate change are therefore widespread, covering both natural and human systems on all continents, posing a threat to the well-being of current and future human beings and other animals.2
With the purpose of influencing decision making on climate change mitigation strategies, scientists have used the concept of a carbon footprint as a tool to assess GHG emissions produced by processes, products, services, individuals, and their consumption patterns, as well as GHG emissions produced within a country, region, or other geographical areas.
Carbon footprint methodology
A methodological framework commonly used to assess environmental impacts of products is the life cycle assessment (LCA).3 Although this method is designed to quantify and analyze various environmental impacts of products (such as climate change, water scarcity, acidification, eutrophication, toxicity, and land use), it is possible to use it exclusively to assess GHG emissions. In this case, LCAs are also known as “carbon footprinting,” and there are specific international guidelines for carrying them out.4
In simple terms, the carbon footprint of a product is estimated by adding all GHG emissions and removals associated with every stage of the product’s life cycle.5 To quantify emissions and removals of different GHGs in a single unit of measure, collected data are transformed to CO2-equivalents (CO2e) by applying a 100-year global warming potential (GWP) factor, which is taken from IPCC’s Assessment Reports. For example, according to the most recent report, the GWP coefficient of methane is 28 CO2e and that of nitrous oxide is 265 CO2e (uncertainty of ±40% and ±30%, respectively, for the 90% confidence interval).
Limitations of carbon footprinting
The methods used to estimate the carbon footprint of products are subject to multiple uncertainties that are expected to be assessed and reported in each specific study.6 Apart from the uncertainty inherent in using quantitative methods to reflect the real world, carbon footprint estimates are subject to uncertainties regarding data,7 as well as uncertainties regarding methodological choices. The use of standards reduces methodological uncertainties by setting specific requirements, and therefore constraining choices. However, at the same time, the standards clearly allow different methodological choices and the use of assumptions.8
Developing LCAs of agricultural products involves distinct challenges given the nature of agricultural systems. One point is that these systems are very diverse, ranging from agronomic and horticultural crops to cattle production systems and aquaculture. They also vary in size and are subject to temporal and spatial variations (e.g., differing regional conditions, and seasonal and annual fluctuations), as well as management techniques that are specific to local conditions. In addition, agricultural systems seem to be dominated by emission sources that cannot be measured directly,9 and it is common that multiple products come from a single system.10 To address these challenges, methodological choices and assumptions are made, giving rise to uncertainties having to do with missing or unavailable data, poor knowledge of underlying processes, and how difficult it often is to verify results.11
Sometimes uncertainties about complex emission sources are so significant that Researchers prefer to exclude them completely from their calculations. That’s the case with land use change impacts, for example, which are intentionally omitted in most LCAs of agricultural products because of lack of information. Since these impacts can be particularly large for some products, special caution is needed when interpreting LCA results (see next section).
Carbon footprint of animal-based and plant-based products12
Although LCAs were originally developed for non-agricultural products, in recent years a growing number of publications have used the LCA framework for estimating the carbon footprint (and other environmental impacts) of agricultural products.13 Given the limitations and weaknesses of LCAs of agricultural products, caution is advised in interpreting results and drawing conclusions. However, when a sufficient amount of LCAs of a particular sector reach similar outcomes, it may be possible to draw reasonable conclusions about environmental impacts.14 This seems to be precisely the case of the animal production sector, where all findings seem to point to large carbon footprints.15
For example, aiming at synthesizing GHG emissions of fresh food products, Clune et al. (2017) reviewed 369 LCAs published between 2000 and 2015. Their systematic literature review resulted in 1,718 carbon footprint estimates for 168 food products, which were converted into the same unit for comparison (i.e., kg CO2e per kg of boneless meat or produce, at the regional distribution center).16 Their results indicate that there are large differences between the carbon footprints of products, and that with exception of milk, carbon footprints of animal products are much larger than carbon footprint of plant products (Table 1 and Figure 1).
Table 1. Carbon footprints of products across broad food categories by Clune et al. (2017) | ||||||
---|---|---|---|---|---|---|
Product | CF estimates (kg CO2e per kg produce or boneless meat) | Number of CF estimates | Number of studies | |||
Mean | Median | Minimum | Maximum | |||
Vegetables (field grown vegetables) | 0.47 | 0.37 | 0.04 | 2.54 | 140 | 33 |
Fruits (field grown fruits) | 0.50 | 0.42 | 0.08 | 1.78 | 250 | 77 |
Cereals | 0.53 | 0.5 | 0.11 | 1.38 | 90 | 31 |
Legumes and pulses | 0.66 | 0.51 | 0.15 | 2.46 | 51 | 16 |
Passive greenhouse fruit and vegetables | 1.02 | 1.1 | 0.32 | 1.94 | 15 | 5 |
Tree nuts combined | 1.42 | 1.2 | 0.43 | 3.77 | 21 | 7 |
Rice | 2.66 | 2.55 | 0.66 | 5.69 | 27 | 12 |
Heated greenhouse fruit and vegetables | 2.81 | 2.13 | 0.84 | 7.4 | 53 | 18 |
Milk | 1.39 | 1.29 | 0.54 | 7.5 | 262 | 77 |
Eggs | 3.39 | 3.46 | 1.30 | 6 | 38 | 19 |
Chicken | 4.12 | 3.65 | 1.06 | 9.98 | 95 | 29 |
Fish (all species combined) | 4.41 | 3.49 | 0.78 | 20.86 | 148 | 47 |
Cream | 5.32 | 5.64 | 2.1 | 7.92 | 4 | 3 |
Pork | 5.85 | 5.77 | 3.2 | 11.86 | 130 | 38 |
Cheese | 8.86 | 8.55 | 5.33 | 16.35 | 38 | 22 |
Butter | 11.52 | 9.25 | 3.7 | 25 | 8 | 4 |
Prawns/shrimp | 14.85 | 7.8 | 5.25 | 38 | 11 | 7 |
Lamb | 27.91 | 25.58 | 10.05 | 56.70 | 56 | 22 |
Beef | 28.73 | 26.61 | 10.74 | 109.5 | 165 | 49 |
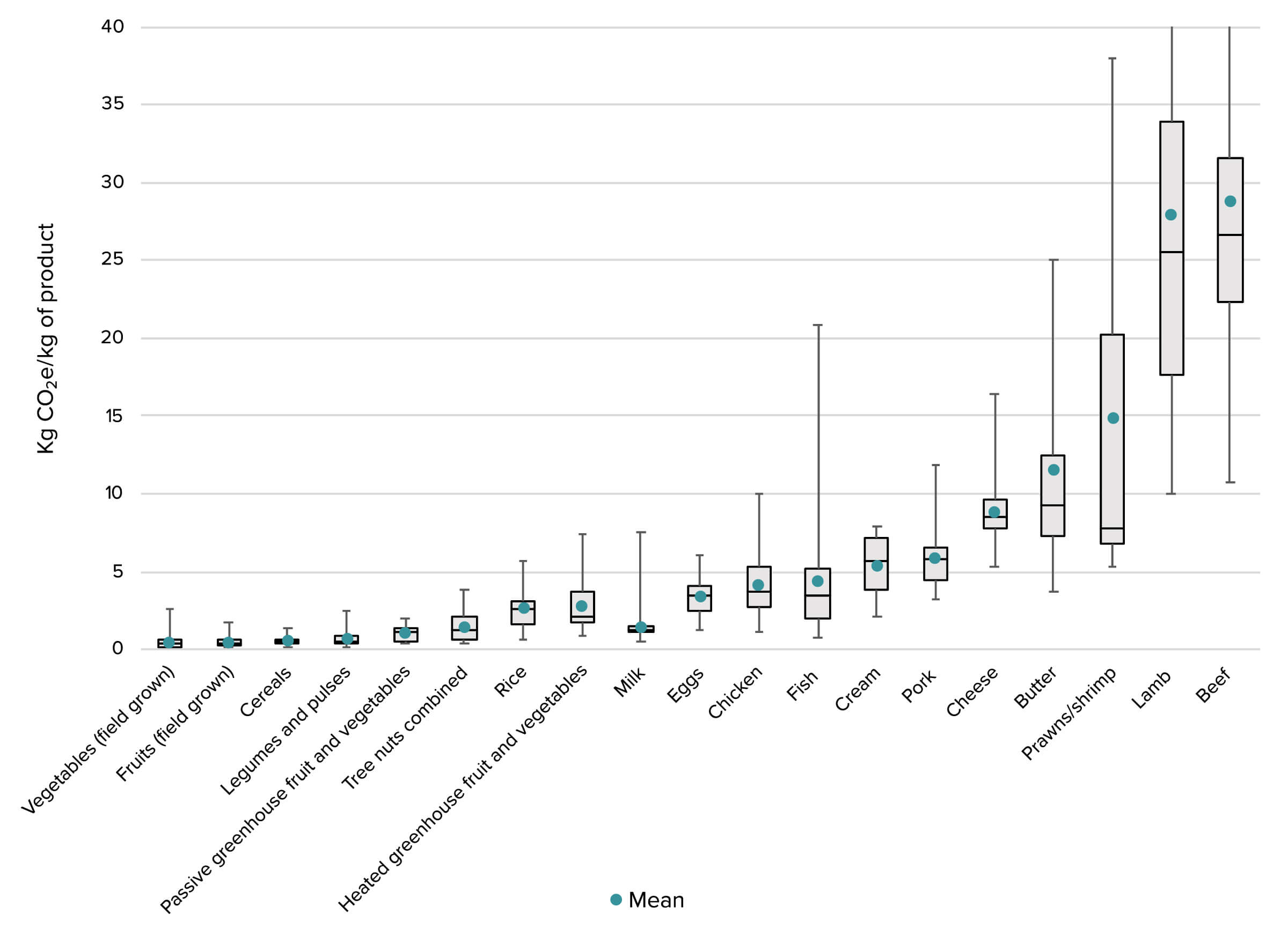
One example of the articles reviewed by Clune et al. (2017) is the study conducted by González et al. (2011), which examined the energy use and GHG emissions associated with the production and transport of common food items to the port of Gothenburg in Sweden. Their analyses were based on results from previous LCA studies, and from their own calculations of carbon footprints of products coming from different countries, in some cases, involving different means of production.17 Their calculations were based on agriculture and processing data obtained from statistics for each country, data of emissions of fuel machinery taken from IPCC guidelines, and data related to fertilizer use and manufacturing obtained from previous studies. In addition, transport distances were determined by using Google Earth. Their results suggest that with exception of vegetables grown in heated greenhouses, carbon footprints of plant-based products are smaller than carbon footprints of animal products (Table 2).18
Table 2. Carbon footprint of products by González et al. (2011) | ||||
---|---|---|---|---|
Product | Carbon footprint estimates (kg CO2e per kg of product) | Number of CF estimates | Number of LCA studies | |
Minimum | Maximum | |||
Tubers, roots and squash | 0.09 | 0.35 | 7 | 2 |
Vegetables (open field grown) | 0.09 | 0.37 | 11 | 1 |
Fruits | 0.16 | 0.55 | 13 | 3 |
Cereals | 0.29 | 1.3 | 14 | 4 |
Legumes | 0.38 | 1.3 | 7 | 3 |
Vegetables (heated greenhouse grown) | 0.75 | 10 | 6 | 2 |
Dairy | 1 | 8.8 | 3 | 3 |
Eggs | 1.6 | 5.5 | 3 | 2 |
Chicken | 2.9 | 6.6 | 2 | 2 |
Pork | 7.2 | 9.2 | 2 | 2 |
Mutton and Lamb | 17 | 36 | 3 | 2 |
Beef | 20 | 40 | 8 | 5 |
One of the reasons why the carbon footprint of animal products is generally larger than the carbon footprint of vegetal products is related to the inefficiencies involved in growing crops to be used as animal feed.19 In fact, feed production is one of the processes contributing to major GHG emissions in animal production. Those emissions mainly come from:20
- The use of fossil fuels in field operations, feed processing and transport, as well as manufacturing of mineral fertilizers (releasing CO2)
- The application of nitrogen fertilizers, crop residues, and deposition of animal manure in feed crops (releasing N2O)21
- Change of land use when forests are cleared to grow feed crops or when feed is cultivated on soils rich in organic matter (releasing CO2).22 Emissions from land use change were excluded by Clune et al. (2017) and González et al. (2011) as is usually the case in LCAs, because of the large uncertainties involved (see below).
Another source of high GHG emissions in animal agriculture is the enteric fermentation23 from feed digestion, which releases methane (CH4). The total carbon footprint of ruminants is usually dominated by substantial CH4 emissions, which explain the exceptionally large carbon footprints of beef and lamb often found in LCA studies.24 FAO’s most recent report on the global environmental impacts of animal agriculture suggests that feed production and enteric fermentation are the two main sources of GHG emissions associated with the animal agriculture sector, accounting for 45% and 39% of sector’s total emissions, respectively.25
Caveat: the omission of land use change impacts
As mentioned above, emissions associated with agriculturally-induced land use change are not usually considered in LCAs of agricultural products, because of the lack of information and the large uncertainties involved.
The omission of indirect land use change impacts may mislead policy recommendations, especially in the case of animal agriculture—which seems to be one of the largest contributors to emissions resulting from land use change.26 For instance, many LCA study results indicate that carbon footprints of ruminants from extensive systems27 are particularly large, given their low feed-conversion efficiencies.28 Based on these results, and with the purpose of reducing GHG emissions from the livestock sector, some studies have favored the production and consumption of animals with higher levels of concentrates29 and cereals in their diet, such as cattle from intensive systems30 as well as pigs and chickens. Since those studies do not consider emissions from indirect land use change, however, it may be the case that replacing extensive systems for intensive systems would lead to increases—rather than decreases—in global GHG emissions. This is because increasing the production of concentrates may lead to increasing the clearance of land to grow feed crops (e.g. cereals, oilseeds, and beans), thereby releasing significant amounts of stored carbon, especially in the case of deforestation in developing countries31
The omission of land use change is only one example of the many considerations that should be taken into account in order to accurately recommend one animal production system over others from a carbon perspective. Since there are genuine scientific uncertainties about the real impacts of animal production systems on climate change, it is advised to be extremely careful before recommending some animal production systems over others on the basis of study results.32
Carbon footprint of animal-based and plant-based diets
Given the apparently substantially larger carbon footprint of animal products in comparison to plant products, it is expected that animal-based diets also have larger carbon footprints than plant-based diets. This hypothesis has been supported by studies that estimate dietary carbon footprints based on carbon footprint estimates of individual food products (i.e., data provided by LCA studies, many of which may be included in Clune et al., 2017) and food consumption or production data.
Consumption data analysis
Scarborough et al. (2014), for example, estimated the carbon footprint of self-selected dietary groups in the U.K. by using consumption data from the EPIC-Oxford Study, and carbon footprint estimates of individual food products consumed in the U.K.33 Their analyses are based on a dataset of more than 55,500 participants who completed a semi-quantitative food-frequency questionnaire (FFQ) that estimates the frequency of consumption of 130 different food products over the previous 12 months. They categorized the subjects in six dietary groups: vegans, vegetarians, fish-eaters, low meat-eaters (>0 and >50 g/d), medium meat-eaters (50 to 99 g/d), and high meat-eaters (>=100 g/d).34 Then they calculated the carbon footprint for each dietary group by using estimates of the carbon footprint of individual products.
Their analysis showed highly statistically significant differences (p<0.0001) in carbon footprints between the six dietary groups, with progressively higher emissions for diets with higher intakes of animal products (Table 3). The largest carbon footprint was found in “high meat-eaters” and the smallest in “vegans,” with the average “high meat” diet having 2.5 times as many GHG emissions than the average “vegan” diet with equivalent energetic content (i.e. 2,000 kcal). Their findings indicate that “dietary GHG emissions associated with self-selected diets in the U.K. are strongly associated with the amount of animal-based products in the diet” (Scarborough et al., 2014, p. 186).
Table 3. Mean GHG emissions per 2,000 kcal by dietary group in the U.K., based on Scarborough et al. (2014) | ||
---|---|---|
Dietary groups | Average carbon footprint (kg CO2e) per day | 95% CIs |
High meat-eaters | 7.19 | 7.16–7.22 |
Medium meat-eaters | 5.63 | 5.61–5.65 |
Low meat-eaters | 4.67 | 4.65–4.70 |
Fish-eaters | 3.91 | 3.88–3.94 |
Vegetarians | 3.81 | 3.79–3.83 |
Vegans | 2.89 | 2.83–2.94 |
Figure 2. Average carbon footprint per day by dietary group in the U.K., based on Scarborough et al. (2014)
It is worth noting that this study does not address uncertainties involved in the estimates of the carbon footprint of individual food products used. Moreover, this study faces limitations associated with the use of consumption data. In particular, estimates of dietary carbon footprints should be taken as underestimates, because they are based on consumption data (rather than production data) and therefore do not consider food wastage. Additionally, FFQ are prone to under-reporting.35
Dietary scenario analysis
Other studies have estimated the difference in carbon footprints between different diets by using modelled reduced-meat dietary scenarios. This method has been used to estimate the effects of dietary choices by changing the amount and/or types of food products. Instead of using self-reported data provided by a single survey/study, this method allows to construct scenarios by using data from different sources (e.g. nutritionists, national dietary guidelines or other study results). Studies that have used this methodology to compare carbon footprints of plant-based and animal-based diets suggest that plant-based diets have smaller carbon footprints than animal-based diets.36
For example, Hallström et al. (2015) reviews 14 journal articles that use dietary scenario analysis to assess environmental impacts of dietary change, including effects in dietary GHG emissions. The articles reviewed varied in terms of their reference scenarios which mostly depended on average per capita consumption data of the studied population, but they also differed in number and kinds of scenarios constructed from the reference scenario. The results of the review indicate that the greatest reduction potential of GHG emissions could be achieved by adopting a vegan diet (25–55%), followed by a vegetarian diet (20–35%), replacing ruminant meat with pork and poultry (20–35%), and eating a “healthy” diet37 (0–35%) (Table 4). The study results suggest that the amount of animal products—as well as the type of animal products—are key factors in dietary carbon footprints.
Table 4. Effect of dietary change on GHG emissions based on Hallström et al. (2015)
Table 4. Effect of dietary change on GHG emissions based on Hallström et al. (2015) | ||||
---|---|---|---|---|
Scenario | Number of scenarios analyzed | Scenario description | Reduction of GHG emissionsa | |
%b | Kg CO2e per yearc | |||
Vegan diet | 6 | All animal products are replaced by plant-based products | 25–55 | 760 (520–1,090) |
Vegetarian diet | 7 | All meat38 is replaced by non-meat products | 20–35 | 540 (110–1,110) |
Ruminant meat replaced by monogastric meat | 2 | All ruminant meat is replaced by pork and poultry | 20–35 | 560 |
Meat partially replaced by plant-based products | 2 | Meat is reduced in favor of plant-based products | +5–0 | +20 (+40–0) |
Meat partially replaced by dairy products | 2 | Meat is reduced in favor of dairy products | 0–5 | 40 (30–50) |
Meat partially replaced by mixed food | 2 | Meat is reduced in favor of a mix of plant-based and dairy products | 0–5 | 80 (40–110) |
Balanced energy intake | 2 | Caloric content is reduced, while composition of diet remains the same | 0–10 | 100 (40–160) |
“Healthy” diet | 14 | Omnivorous diet based on different dietary guidelines | 0–35 | 210 (+40–490) |
a “+” indicates an increase, rather than a decrease, in GHG emissions.
b Percentage of reduction in GHG emissions compared to average consumption data.
c Average effect (minimum change – maximum change).
However, none of the reviewed articles included GHG emissions produced by indirect land use change, which might suggest an overestimation of potential reduction of GHG emissions by replacing ruminant meat with pork and poultry meat (as discussed above).
One example of the articles reviewed by Hallström et al. (2015) is the study conducted by Berners-Lee et al. (2012) which assesses the impacts of dietary change in GHG emissions for the U.K. They take the U.K. average per capita food supply from FAOSTAT39 as a reference scenario and modify it with selected substitutes to create six dietary scenarios: three vegetarian and three vegan scenarios (Table 5).
Table 5. GHG emissions embodied in different diets per day in the U.K. (based on Berners-Lee et al., 2012) | |||
---|---|---|---|
Dietary scenario | Type | Description | GHG emissions (kg CO2e) per day |
Baseline | Omnivorous | Average per capita U.K. food supply of 3,458 kcal per day | 7.40 |
Scenario 1 | Vegetarian | U.K. average diet with meat energy replaced by dairy energy* | 5.79 |
Scenario 2 | Vegetarian | U.S. average vegetarian diet* | 6.06 |
Scenario 3 | Vegetarian | U.K. average diet with meat energy replaced by “healthy” non-dairy alternatives* | 5.54 |
Scenario 4 | Vegan | U.K. average diet with meat and dairy energy replaced by plant-based alternatives* | 5.14 |
Scenario 5 | Vegan | U.S. vegan diet* | 5.68 |
Scenario 6 | Vegan | U.K. average diet with meat and dairy energy replaced by “healthy” plant-based alternatives* | 5.55 |
* Normalized to U.K. food supply per capita energy
Their estimations indicate that changing from an omnivorous diet to a vegetarian diet would reduce individual carbon footprint by 22%, while changing to a vegan diet would reduce it by 26%. However, calculations of GHG emissions used in this study did not consider the effects of land use change in GHG emissions; otherwise, the reduction in the carbon footprint by adopting a vegan diet could have been substantially greater.40
In conclusion, results from LCA studies of agricultural products and from estimations of the carbon footprint of different diets suggest that animal-based products and diets have generally larger carbon footprints than plant-based products and diets. Estimates of the carbon footprint of products indicate that despite the great variability among agricultural products, animal products are generally more carbon-intensive than plant-based products, even without considering the emissions produced by land use change. This is partly because of the inefficiencies involved in growing crops and pastures to be used as animal feed, together with emissions produced by enteric fermentation and animal waste. Since the carbon footprint of diets is estimated on the basis of data provided by LCA of products, it is no surprise that estimates of dietary carbon footprints follow the same pattern as the carbon footprint of agricultural products. Different methodologies used, as well as varying data from different locations, reach the same conclusion: plant-based diets are more climate-friendly than animal-based diets.
Water Footprint of Diet Choices
Freshwater, in sufficient quantities and appropriate quality, is a vital resource for the survival and well-being of humans and many other animals. Estimates suggest that around 30% of the world’s population lives in areas facing water scarcity,41 and that 66% of the world’s population lives under severe water scarcity at least one month per year.42 With current production and consumption patterns, the pressure on limited freshwater resources is likely to become more intense over the years43 Along with growing water demand, climate change is creating additional pressure on water resources, as the variability of precipitation patterns and the frequency of droughts and floods are increasing.44
Aiming at influencing decision making on water sustainability, scientists and policy-makers have used the concept of the “water footprint” as a tool to assess freshwater use associated with processes, products, individuals, and geographical areas. The concept of the water footprint was coined by Hoekstra and Hung (2002) and further developed in the Water Footprint Assessment Manual by Hoekstra et al. (2011). There, the water footprint is defined as “a comprehensive indicator of freshwater appropriation by humans,” specified in relation to time and location, including both direct and indirect water consumption and pollution.45
Water footprint methodology
The Water Footprint Assessment Manual includes a detailed description of the methodology for quantifying the water footprint of products along the full life cycle. In general terms, this methodology suggests that the water footprint of a product is to be calculated by adding the blue, green and grey water footprints of all the process steps involved in the production and supply chain of the product. The three types of water footprints are defined by Hoekstra et al. (2011) as follows:
- Blue water footprint: volume of surface and groundwater (blue water) consumed during the production process (including evaporated water, the water that is incorporated into a product along the supply chain, and the water that does not return to the same catchment area or in the same period of time).
- Green water footprint:46 volume of rainwater (green water) consumed during the production process. It only includes the rainwater that is temporarily stored in the soil or vegetation (therefore excluding the water that becomes runoff). In products based on crops, it refers to the evaporation and transpiration from fields and plantations plus the (green) water incorporated into the crop.
- Grey water footprint: volume of polluted water. It is defined as the volume of water that is required to assimilate pollutants based on ambient water quality standards.
Nevertheless, the Water Footprint Assessment Manual, which was published by the Water Footprint Network (WFN), is not the only methodological approach for water footprinting. The concept of water footprint has also become popular among the Life Cycle Assessment (LCA) community, who have integrated water use into the LCA methodological framework,47 developing a specific international standard for water footprint estimations (ISO 14046). This methodological approach differs in various respects from the one previously suggested by WFN, giving rise to a debate between the two communities.48 Despite discrepancies between the two approaches, it’s been suggested that they should be perceived as complementary, rather than as competing methodologies, since they are useful for different purposes.49 Moreover, studies have suggested that when using similar data sources, the two methods can lead to water footprint results that, despite their differences in methodology, are somewhat consistent between each other.50 Without intending to take sides in this debate and hoping that water footprint estimates are consistent between both methodologies, this report only includes water footprint estimates based on the WFN methodology. Therefore, they are expressed in volumetric units, and include blue, green and/or grey water footprints. We made this choice basically because our literature search indicated that WFN guidelines are more popular than the LCA approach among studies that compare the water footprint of plant-based and animal-based food products and diets.
Limitations of water footprinting
It is recognized that uncertainties are not properly analyzed and reported in water footprint assessments,51 which implies that caution is needed when interpreting the results. Although uncertainty and sensitivity analyses are not popular among water footprint assessments, uncertainties involving data are normally acknowledged. Data quality issues seem to be particularly common, since a large number of specific data (in terms of location and time) is required to estimate accurately the water footprint of a product.52 In many cases, however, specific data is lacking, and estimates are made on the basis of averages.53
Water footprint of animal-based and plant-based products
Attempts to assess the water footprint of food products have found great differences between types of products, with animal products being known to be particularly water-intensive.54 The assessment of water footprints of selected agricultural products compiled by Mekonnen and Hoekstra (2012) indicates that at a global level, compared to vegetal products, animal products have generally larger water footprints not only per mass but also per calorie (Table 6 and Figure 3).
Table 6. Global average water footprints of selected vegetal and animal products for the period 1996–2005 (based on Mekonnen & Hoekstra, 2012) | ||||||
---|---|---|---|---|---|---|
Food Item | Water footprint per ton (m3/ton) | Calorie content (kcal/kg) | Water footprint per calorie (L/kcal) | |||
Green | Blue | Grey | Total | |||
Sugar crops | 130 | 52 | 15 | 197 | 285 | 0.69 |
Vegetables | 194 | 43 | 85 | 322 | 240 | 1.34 |
Starchy roots | 327 | 16 | 43 | 386 | 827 | 0.47 |
Fruits | 726 | 147 | 89 | 962 | 460 | 2.09 |
Cereals | 1,232 | 228 | 184 | 1,644 | 3,208 | 0.51 |
Oil crops | 2,023 | 220 | 121 | 2,364 | 2,908 | 0.81 |
Pulses | 3,180 | 141 | 734 | 4,055 | 3,412 | 1.19 |
Nuts | 7,016 | 1,367 | 680 | 9,063 | 2,500 | 3.63 |
Milk | 863 | 86 | 72 | 1,021 | 560 | 1.82 |
Eggs | 2,592 | 244 | 429 | 3,265 | 1,425 | 2.29 |
Chicken meat | 3,545 | 313 | 467 | 4,325 | 1,440 | 3 |
Butter | 4,695 | 465 | 393 | 5,553 | 7,692 | 0.72 |
Pig meat | 4,907 | 459 | 622 | 5,988 | 2,786 | 2.15 |
Sheep meat | 8,253 | 457 | 53 | 8,763 | 2,059 | 4.25 |
Bovine meat | 14,414 | 550 | 451 | 15,415 | 1,523 | 10.19 |
Figure 3. Global average water footprint of selected vegetal and animal products for the period 1996–2005 (based on Mekonnen & Hoekstra, 2012)
According to their results, there is a great variation between the water footprint of products, with sugar crops having the smallest total water footprint (197 m3/ton) and beef having the largest (15415 m3/ton) (Figure 3). In terms of the water footprint per calorie, starchy roots and cereals seem to be the most water efficient while beef the least efficient; it takes about 20 times more water to produce one calorie of beef than to produce one calorie of cereal. Therefore, they conclude that, in general, it is more water-efficient to obtain calories from vegetal products than animal products.55 The clearest exception is the water footprint of nuts, which is particularly large, not only in total terms but also in delivering calories.
We should note that the grey water footprint estimated in Mekonnen and Hoekstra (2012) only included the pollution caused by nitrogen fertilizers, excluding potential pollution by pesticides, other fertilizer ingredients, manure, heavy metals, and antibiotics. Their estimates of the grey water footprint also exclude the nitrogen leaching and runoff from manure, which may be especially significant in intensive systems of animal production where great quantities of animal waste are deposited in freshwater systems. Therefore the authors state that their values of grey water footprints should be taken as underestimates, especially in the case of animal products.
In the case of animal products, most of their water footprint corresponds to the first step of the supply chain, i.e., growing the feed for animals. Pastures and growing crops to feed farmed animals consume large volumes of water resources through evapotranspiration, irrigation, and pollution by fertilizers, accounting for most of the total water footprint for final animal products. Mekonnen and Hoekstra (2012) estimated that 98% of the total water footprint of animal production corresponds to animal feed, most of which is grown in different areas from where consumption occurs. The other 2% corresponds to drinking water, service water (water used to clean facilities, wash animals, and waste disposal) and feed-mixing water. The water footprint of animal products, therefore, mainly refers to water consumed or polluted to produce animal feed.
Based on Mekonnen and Hoekstra’s (2012) estimates, Gerbens-Leenes et al. (2013) show some general trends in the water footprint of animal products and identify three driving factors:
- Feed conversion efficiency. It refers to the quantity of feed needed to produce a certain amount of meat, eggs and milk. It normally improves from grazing systems to intensive systems, because animals in intensive systems are bred to grow faster and killed younger, eat more feed concentrates (instead of roughages) and move considerably less.
- Feed composition. It refers to the composition of feed consumed by the animals in each system, and it works in the opposite direction: it favors grazing systems over intensive systems. Since animal feed concentrates have larger water footprints than roughages,56 and animals raised in intensive systems eat more feed concentrates, there is an increase of the water footprint in intensive systems with respect to feed composition.
- Feed origin. This factor cannot be ignored, especially because water use related to feed crop production varies across regions, depending on climatic conditions and agricultural practices.57
Water footprint of milk and burger vs soy substitutes
Ercin et al. (2012) assessed the water footprints of soy milk and soy burger from two specific locations (a factory in Belgium and a factory in the Netherlands), and compared them with estimates of the water footprint of cow’s milk and beef burger from various countries. Their results indicate that the animal origin version has a larger water footprint than the soy version, both in the case of milk and burgers. In particular, they found that one liter of cow’s milk requires about 3.5 times the volume of water required to produce one liter of soy milk, and that one 150g beef burger requires about 13 times the volume of water required to produce one 150g soy burger (Table 7).
Table 7. Water footprint of soy milk and soy burger, and cow’s milk and beef burger (based on Ercin et al., 2012) | ||
---|---|---|
Food item | Water footprint of soy product | Water footprint of cow product |
Milk (1 L) | 297 L | 1,050 L |
Burger (150 g) | 158 L | 2,350 L |
Water footprint of animal-based and plant-based diets
According to Hoekstra and Mekonnen (2012), food production is by far the largest water user: about 92% of our global freshwater footprint corresponds to agriculture, 4.4% to industrial water use, and 3.6% to domestic water use. If those estimates were correct, shifting our diets would have a great potential to influence the overall water footprint of individuals.
Some studies have estimated the water footprint of different diets, and results seem to be in line with tendencies in water footprints of individual agricultural products. In general, plant-based diets seem to have smaller water footprints than animal-based diets.
For example, results from Jalava et al. (2014) indicate that plant-based diets have the greatest potential to reduce the blue and green water footprints of global consumption. They created a reference scenario based on food supply data from FAO Food Balance Sheets that was adjusted to dietary guidelines (dietary energy requirement from FAO and macronutrient intake from WHO). Then they created four dietary scenarios, progressively reducing animal products, and estimated the blue and green water footprints for each scenario, based on water footprint estimates from Mekonnen and Hoekstra (2011). Their results indicate that water footprints were smaller as consumption of animal products was lower (Table 8).
Table 8. Global water use for dietary scenarios with different amounts of animal-based protein intake at global level (based on Jalava et al. 2014) | |||
---|---|---|---|
Dietary scenarios | Scenario description | Blue water use (% difference to recommended diet) | Green water use (% difference to recommended diet) |
Recommended diet | Based on FAO and WHO dietary guidelines | 0% | 0% |
A50 | 50% of animal protein intake | -3.80% | -6% |
A25 | 25% of animal protein intake | -6.40% | -11% |
A12.5 | 12.5% of animal protein intake | -8.90% | -15.10% |
A0 | 0% of animal protein intake | -14.40% | -20.80% |
The same pattern seems to be supported by the results found in Vanham et al. (2013). They estimated for the four E.U. zones the water footprint of three dietary scenarios: current diet, “healthy” diet (based on regional dietary guidelines), and vegetarian diet (meat-free diet including dairy and eggs). Their results indicate that vegetarian diets would have the lowest water footprints for each E.U. zone (Table 9 and Figure 4).
Table 9. Water footprint of dietary scenarios for the four E.U. zones (based on Vanham et al., 2013) | ||||
---|---|---|---|---|
Dietary scenario | Water footprint (L per capita per day) per E.U. zone* | |||
South | East | West | North | |
Current diet | 5875 | 4053 | 3761 | 3197 |
“Healthy” diet | 4,110 (-30%) | 3,606 (-11%) | 2,766 (-26%) | 3,091 (-3%) |
Vegetarian diet | 3,476 (-41%) | 2,956 (-27%) | 2,208 (-41%) | 2,166 (-32%) |
* (% difference to current diet)
Figure 4. Water footprint of dietary scenarios for the four E.U. zones (based on Vanham et al., 2013)
A more recent study by Vanham et al. (2016) presents an analysis of the water footprint of three diet scenarios for 13 Mediterranean cities. The reference scenario was based on food supply data from FAO Food Balance Sheets for the period 1996-2005 as well as data from food and nutrition surveys for each country. Besides, three scenarios were developed for each city based on recommended dietary guidelines (especially on the traditional Mediterranean diet which is known as “healthy”, and Turkish dietary guidelines were used for Turkish cities), but with differences in the consumption of animal products:
- “Healthy” meat diet: Mediterranean diet with meat
- “Healthy” pesco-vegetarian diet: Identical to “healthy” meat diet, but meat products are replaced by fish, cereals and pulses with the same amount of calories and protein.
- “Healthy” vegetarian diet: identical to “healthy” pesco-vegetarian diet but all fish products are replaced by pulses and cereals with the same amount of calories and protein.
Their results suggest that the “healthy” vegetarian diet leads to the greatest reduction in the water footprint of consumption for every city (a reduction of 30% to 53% with respect to current diets) (Table 10 and Figure 4). It should be noted that values of the water footprint of this study refer to blue and green water footprint only.
Table 10. Water footprint of dietary scenarios for 13 Mediterranean cities (based on Vanham et al., 2016) | |||||
---|---|---|---|---|---|
Country | City | Water footprint (L/cap/day) of dietary scenarios | |||
Reference | “Healthy” Meat Diet | “Healthy” Pesco Vegetarian Diet | “Healthy” Vegetarian Diet | ||
Slovenia | Ljubljana | 3,277 | 2,459 | 2,309 | 2,211 |
France | Lyon | 3,835 | 2,363 | 2,163 | 2,076 |
Turkey | Istanbul | 4,316 | 4,115 | 2,716 | 2,557 |
Turkey | Ankara | 4,323 | 4,115 | 2,711 | 2,552 |
Croatia | Dubrovnik | 4,537 | 3,654 | 3,283 | 3,194 |
Italy | Bologna | 4,933 | 2,875 | 2,604 | 2,518 |
Italy | Reggio Emilia | 4,933 | 2,872 | 2,601 | 2,515 |
Italy | Genova | 4,935 | 2,882 | 2,611 | 2,524 |
Greece | Athens | 5,017 | 3,170 | 2,853 | 2,752 |
Italy | Pisa | 5,157 | 2,796 | 2,526 | 2,438 |
Spain | Manresa | 5,441 | 3,579 | 3,304 | 3,183 |
Spain | Zaragoza | 5,441 | 3,580 | 3,306 | 3,184 |
Figure 5. Water footprint of dietary scenarios for 13 Mediterranean cities (based on Vanham et al., 2016)
In conclusion, results from studies of agricultural products and from estimations of the water footprint of different dietary scenarios suggest that farmed animal products tend to have larger water footprints that vegetal products, and that vegetarian diets seem to have the greatest potential to reduce dietary water footprints. Water footprint estimates indicate that despite the great variability between products, animal products tend to be more water-intensive than vegetal products regarding the three components of the water footprint (green, blue and grey), even without considering many animal sources of freshwater pollution. Since the water footprint of diets is estimated on the basis of data from the water footprint of products, studies that use dietary scenario analyses also suggest that vegetarian diets are more water-friendly than omnivorous diets.
Dietary groups,Average carbon footprint (kg CO2e) per day High meat-eaters,7.19,1 Medium meat-eaters,5.63,1 Low meat-eaters,4.67,1 Fish-eaters,3.91,1 Vegetarians,3.81,1 Vegans,2.89,1
Products,Green Water Footprint,Blue Water Footprint,Grey Water Footprint Sugar crops,130,52,15 Vegetables,194,43,85 Starchy roots,327,16,43 Fruits,726,147,89 Cereals,1232,228,184 Oil crops,2023,220,121 Pulses,3180,141,734 Nuts,7016,1367,680 Milk,863,86,72 Eggs,2592,244,429 Chicken meat,3545,313,467 Butter,4695,465,393 Pig meat,4907,459,622 Sheep meat,8253,457,53 Bovine meat,14414,550,451
Products,Current diet,“Healthy” diet,Vegetarian diet South,5875,4110,3476 East,4053,3606,2956 West,3761,2766,2208 North,3197,3091,2166
City,Reference,“Healthy” Meat Diet,“Healthy” Pesco Vegetarian Diet,“Healthy” Vegetarian Diet Jerusalem,5789,3285,2805,2708 Manresa,5441,3579,3304,3183 Zaragoza,5441,3580,3306,3184 Pisa,5157,2796,2526,2438 Athens,5017,3170,2853,2752 Genova,4935,2882,2611,2524 Bologna,4933,2875,2604,2518 Reggio Emilia,4933,2872,2601,2515 Dubrovnik,4537,3654,3283,3194 Ankara,4323,4115,2711,2552 Istanbul,4316,4115,2716,2557 Lyon,3835,2363,2163,2076 Ljubljana,3277,2459,2309,2211
According to most recent IPCC report: “Anthropogenic greenhouse gas emissions have increased since the pre-industrial era, driven largely by economic and population growth, and are now higher than ever. This has led to atmospheric concentrations of carbon dioxide, methane and nitrous oxide that are unprecedented in at least the last 800,000 years. Their effects, together with those of other anthropogenic drivers, have been detected throughout the climate system and are extremely likely to have been the dominant cause of the observed warming since the mid-20th century.” (IPCC, 2014)
FAO’s report on climate change and animal agriculture states: “Climate change is transforming the planet’s ecosystems and threatening the well-being of current and future generations.” (Gerber et al., 2013)
LCA is a quantitative method used to determine the environmental impacts across the whole supply chain, for which guidelines are provided by the ISO standards (ISO 14040 and ISO 14044). Conducting a LCA comprises four general phases: goal and scope definition (i.e., determining the goal of the study, the system boundaries and the functional unit), inventory analysis (i.e., gathering and verifying data), impact assessment, and interpretation of results. Despite their well-recognized limitations, LCA is a tool that helps to identify where the main impacts lie in the life cycle of a product, pointing to where remedial action is needed.
The Product Standard by the GHG Protocol, PAS 2050 by the British Standard Institution, and ISO 14067. These standards are broadly consistent in their quantification methods, as they are based on the methodological framework set by existing ISO standards, despite slight differences in purpose and development processes.
Five general life cycle stages are typically identified: materials acquisition and pre-processing, production/manufacture, product distribution and storage, product use, and final disposal/recycling. Emissions from the last two stages (i.e., product use and final disposal) are excluded in partial (cradle-to-gate) LCAs, while they are normally included in complete (cradle-to-grave) LCAs.
A guideline for communicating different types of uncertainties related to carbon footprinting can be found in the Product Standard. Unfortunately, uncertainty analyses are not common among LCAs of agricultural products.
Data on GHG emissions and removals of a process can be collected either by directly measuring or modelling the emissions/removals through different techniques, or by collecting activity data and emission factors, which are then multiplied. According to the Product Standard, not only direct GHG emissions/removals data (i.e., directly measured or modelled data on emissions released from a process or removals absorbed from the atmosphere), but also process activity data (i.e., quantitative measures of a process that results in GHG emissions/removals) and emission factors data (i.e., GHG emissions per unit of activity data) are subject to uncertainties that are supposed to be reported in every assessment.
For example, choices regarding allocation methods (i.e., the method selected to divide total GHG emissions/removals resulting from a process between their multiple inputs or outputs), assumptions regarding the use of the product (e.g. refrigeration, preparation) and end-of-life processes (e.g. land filling, incineration, recycling).
Nonpoint emission sources, which refer to emissions that cannot be attributed to end of pipe or end of stack emissions, and therefore cannot be measured directly, dominate agricultural production (Caffrey & Veal, 2013)
Röös, E., Tidåker, P., Strid, I., & Hansson, P. (2013). Can carbon footprint serve as an indicator of the environmental impact of meat production? Ecological Indicators, 24, 573-581. https://www.sciencedirect.com/science/article/pii/S1470160X12002956
The literature search for this report was completed in March 2018 across two types of literature: peer reviewed scholarly journal papers, which were searched on Sciencedirect, and reports from recognized international institutions and other environmentalist organizations. By scanning the reference list of the initial studies reviewed, we identified additional studies, and then selected as key references for our analyses relevant publications for comparing plant-based and animal-based products from a general point of view, such as systematic literature reviews, meta-analyses of LCAs, and regional and global reports.
Examples of meta-publications of LCAs of agricultural products are:
– Clune, S., Crossin, E., Verghese, K. (2017). Systematic review of greenhouse gas emissions for different fresh food categories. Journal of Cleaner Production, 140, 766-783. https://www.sciencedirect.com/science/article/pii/S0959652616303584
– Nijdam, D., Rood, T., & Westhoek, H. (2012). The price of protein: Review of land use and carbon footprints from life cycle assessments of animal food products and their substitutes. Food Policy, 37, 760-770. https://www.sciencedirect.com/science/article/pii/S0306919212000942
– Yan, M., Humphreys, J., & Holden, N. (2011). An evaluation of life cycle assessment of European milk production. Journal of Environmental Management, 92, 372-379. https://www.ncbi.nlm.nih.gov/pubmed/21055870
– de Vries, M., & de Boer, I. J. M. (2010). Comparing environmental impacts for livestock products: A review of life cycle assessments. Livestock Science, 128, 1-11. https://www.sciencedirect.com/science/article/pii/S1871141309003692
– Roy, P., Nei, D., Orisaka, T., Xu, Q., Okadome, H., Nakamura, N., & Shiina, T. (2009). A review of life cycle assessments (LCA) on some food products. Journal of Food Engineering, 90, 1-10. https://www.sciencedirect.com/science/article/pii/S0260877408002793
– Pelletier, N. (2007). Environmental performance in the US broiler poultry sector: Life cycle energy use and greenhouse gas, ozone depleting, acidifying and eutrophying emissions. Agricultural Systems, 98, 67-73. https://www.sciencedirect.com/science/article/pii/S0308521X08000401See Garnett, T. (2008). Cooking up a storm: Food, greenhouse gas emissions and our changing climate. Food Climate Research Network. https://www.fcrn.org.uk/fcrn/publications/cooking-up-a-storm
Examples of studies that find animal products to be substantial contributors to GHG emissions:
– Clune, S., Crossin, E., Verghese, K. (2017). Systematic review of greenhouse gas emissions for different fresh food categories. Journal of Cleaner Production, 140, 766-783. https://www.sciencedirect.com/science/article/pii/S0959652616303584
– Nijdam, D., Rood, T., & Westhoek, H. (2012). The price of protein: Review of land use and carbon footprints from life cycle assessments of animal food products and their substitutes. Food Policy, 37, 760-770. https://www.sciencedirect.com/science/article/pii/S0306919212000942
– González, A. D., Frostell, B., & Carlsson-Kanyama, A. (2011). Protein efficiency per unit energy and per unit greenhouse gas emissions: Potential contribution of diet choices to climate change mitigation. Food Policy, 36, 562-570. https://www.sciencedirect.com/science/article/pii/S030691921100090X
– Audsley, E., Brander, M., Chatterton, J., Murphy-Bokern, D., Webster, C., and Williams, A. (2009). How low can we go? An assessment of greenhouse gas emissions from the UK food system and the scope to reduce them by 2050. WWF-UK. https://www.fcrn.org.uk/fcrn/publications/how-low-can-we-go
– Garnett, T. (2008). Cooking up a storm: Food, greenhouse gas emissions and our changing climate. Food Climate Research Network. https://www.fcrn.org.uk/fcrn/publications/cooking-up-a-storm
– Carlsson-Kanyama, A., & González, A. D. (2007). Non-CO2 greenhouse gas emissions associated with food production: methane (CH4) and nitrous oxide (N2O). KTH report Trita-IMA 2007: 22. http://citeseerx.ist.psu.edu/viewdoc/download?doi=10.1.1.468.2795&rep=rep1&type=pdf
– Williams, A. G., Audsley, E., & Sandars, D. L. (2006). Determining the environmental burdens and resource use in the production of agricultural and horticultural commodities. Main Report. Defra Research Project IS0205. Bedford: Cranfield University and Defra. http://randd.defra.gov.uk/Default.aspx?Module=More&Location=None&ProjectID=11442Clune et al. (2017) do not specify what GWP factors were used in their analyses, probably because many of the LCAs reviewed did not do it either. Therefore, we can assume that carbon footprint estimates were not adjusted to the same GWP factors, and that values would change if methane and nitrous oxide were converted in CO2e using the factors provided by the most recent IPCC report.
Like Clune et al. (2017), González et al. (2011) do not specify what GWP factors were used in their own calculations nor in the carbon footprint estimates taken from other studies. Hence, we can also assume that estimates are not adjusted to the same GWP factors.
González et al. (2011) also compared animal products and vegetal products in terms of protein delivery efficiencies. The study results suggest that vegetal products are much more efficient in delivering protein than animal products, regarding both energy use and GHG emissions.
Röös, E., Tidåker, P., Strid, I., & Hansson, P. (2013). Can carbon footprint serve as an indicator of the environmental impact of meat production? Ecological Indicators, 24, 573-581. https://www.sciencedirect.com/science/article/pii/S1470160X12002956
N2O emissions represent about half of feed emissions according to Gerber et al. (2013)
Land use change also releases significant amounts of N2O when new land use is associated with application of nitrogen fertilizers, as suggested by Neill et al. (2005).
Enteric fermentation is fermentation that takes places in the stomach/rumen of animals, where microorganisms break down carbohydrates into simple molecules that can be digested by the animal. This process releases methane, and ruminants produce larger amounts of methane than monogastric animals (Gerber et al., 2013)
Examples of reviews of LCA studies that found ruminant products having very large carbon footprints are those conducted by Clune et al. (2017), Nijdam et al. (2012), González et al. (2011), de Vries and de Boer (2010), and Roy et al. (2009).
According to Audsley et al. (2009), cattle production may contribute to at least 85% of all land use change emissions for food production in the U.K.
Extensive agricultural systems are systems that use small amounts of labor and capital in relation to area of land being farmed, whereas intensive systems use large amounts of labor and capital relative to land area (Encyclopedia Britannica). In the context of animal agriculture, extensive systems are usually large pastures for ruminant meat production, while intensive systems refer to industrial units for dairy, pig, and chicken production.
For example, Nijdam et al. (2012) suggest that carbon footprints of beef from extensive production systems are larger than carbon footprints of beef from intensive production systems. Moreover, results by Nijdam et al. (2012), González et al. (2011), de Vries and de Boer (2010), and other studies have consistently suggested that carbon footprints of ruminants are often larger than carbon footprints of monogastric animals such as pigs and chickens.
FAO defines concentrates as: “feeds that contain a high density of nutrients, usually low in crude fibre content (less than 18% of dry matter (DM)) and high in total digestible nutrients.” Concentrates are typically mixtures of cereals (mostly corn), oilseeds, and soybeans.
For example:
Swain, M., Blomqvist, L., McMara, J., & Ripple, W. J. (2018). The environmental impact of global diets. Science of the Total Environment, 610-611, 1207-1209. http://trophiccascades.forestry.oregonstate.edu/sites/trophic/files/Swain2017.pdfThese considerations are further developed by Garnett et al. (2017), Garnett (2008) and de Vries and de Boer (2010).
Scarborough, P., Appleby, P. N., Mizdrak, M., Briggs, A. D. M., Travis, R. C., Bradbury, K. E., & Key, T. J. (2014). Dietary greenhouse gas emissions of meat-eaters, fish-eaters, vegetarians and vegans in the UK. Climatic Change, 125, 179-192.
The dietary groups selected by Scarborough et al. (2014) suggest that fish-eaters are not meat-eaters, a characterization which we feel is incorrect.
Becker, W., Welten, D. (2001). Under-reporting in dietary surveys – implications for development of food-based dietary guidelines. Public Health Nutrition, 4, 683–687. https://www.ncbi.nlm.nih.gov/pubmed/11683562
For example:
– Hallström, E., Carlsson-Kanyama, A., & Börjesson, P. (2015). Environmental impact of dietary change: a systematic review. Journal of Cleaner Production, 91, 1-11. https://www.sciencedirect.com/science/article/pii/S0959652614012931
– Werner, L. B., Flysjö, A., & Tholstrup, T. (2014). Greenhouse gas emissions of realistic dietary choices in Denmark: The carbon footprint and nutritional value of dairy products. Food & Nutrition Research, 58. http://dx.doi.org/10.3402/fnr.v58.20687
– Berners-Lee, M., Hoolohan, C., Cammack, H., & Hewitt, C. (2012). The relative greenhouse gas impacts of realistic dietary choices. Energy Policy, 43, 184–190. https://www.sciencedirect.com/science/article/pii/S0301421511010603
– Baroni, L., Cenci, L., Tettamanti, M., & Berati, M. (2007). Evaluating the environmental impact of various dietary patterns combined with different food production systems. European Journal of Clinical Nutrition, 61, 279-286. https://www.ncbi.nlm.nih.gov/pubmed/17035955“Healthy” diet is the name given by Hallström (2015) to scenarios that are based on national and international nutritional guidelines, such as the Dutch Dietary Guidelines, the Nordic Nutrition Recommendations, the Federation for Independent Health Consultation, and WHO/FAO. It’s worth noting that ACE does not endorse those diets as necessarily healthy.
We assume that by “all meat” Hallström et al. (2015) refer to all types of meat, including fish as well; unfortunately, they do not specify it.
FAOSTAT. (2011). Food balance sheet for the U.K. for 2007. http://www.fao.org/faostat/en/#data/FBS
As suggested by Audsley et al. (2009), who suggest that including CO2 emissions resulting from U.K. food-consumption induced land use change increases food’s carbon footprint by 50%.
Here we use water scarcity as referring to physical water scarcity, rather than economic water scarcity. Physical water scarcity occurs when physically there are no enough freshwater resources to meet the demand, whereas economic water scarcity occurs when there is no enough investment in water infrastructure (and workforce) to meet the demand, independently of the amount of freshwater resources physically available. Examples of estimates of physical water scarcity at a global level:
– Kummu et al. (2010): 35% of the world’s population lives in chronic water shortage (i.e. less than 1000 m3/capita/year).
– Islam et al. (2007): 25% of the world’s population lives below 500 m3/capita/year.Mekonnen & Hoekstra (2016) calculate water scarcity as the ratio between blue water footprint and blue water availability (on a monthly basis, at a local level). Results of water scarcity are categorized in four categories: low (WS<1.0), moderate (1.0<WS<1.5), significant (1.5<WS<2.0) and severe (WS>2.0).
Jalava et al. (2014). Diet change—a solution to reduce water use? Environmental Research Letters, 9. doi:10.1088/1748-9326/9/7/074016
IPCC. (2014). Climate Change 2014: Synthesis Report. Contribution of Working Groups I, II and III to the Fifth Assessment Report of the Intergovernmental Panel on Climate Change [Core Writing Team, R. K. Pachauri and L. A. Meyer (eds.)]. Geneva, Switzerland: IPCC. Retrieved from http://www.ipcc.ch/report/ar5/syr/
Hoekstra, A. Y., Chapagain, A. K., Aldaya, M. M., & Mekonnen, M. M. (2011). The Water Footprint Assessment Manual: Setting the Global Standard. London: Earthscan. Retrieved from http://waterfootprint.org/media/downloads/TheWaterFootprintAssessmentManual_2.pdf
Although blue water resources have higher opportunity costs than green water resources, Hoekstra et al. (2011) argues that it is important to include green water resources in water footprint estimations because of their limited availability. In agriculture, green and blue water resources can be substituted for each other (in physical terms) so that a more complete picture of the water footprint can be obtained by counting them both.
LCA is a quantitative method used to determine the environmental impacts across the whole supply chain, for which guidelines are provided by the International Organization for Standardization.
There has been an exchange of letters between the LCA community and the WFN community: letter from Pfister and Hellweg (2009); reply from Hoekstra et al. (2009); letter from Ridoutt and Huang (2012); reply from Hoekstra and Mekonnen (2012). There was also a collaborative attempt to clarify differences between methodologies (Boulay et al., 2013) followed by a letter from Pfister and Ridoutt (2014). More recently a critique from Hoekstra (2016) was published, followed by a reply from Pfister et al. (2017).
The WFN’s approach only focuses on water related impacts, while the LCA’s approach focuses on many environmental impacts of products (e.g., climate change, water use, land use, toxicity, eutrophication), being water use just one impact among many. This is partly why according to the WFN’s approach, the water footprint is expressed in terms of volume of water used, and includes blue, green and grey water footprints, whereas according to the LCA community, the water footprint is expressed in terms of water scarcity metrics (e.g. water stress index) and only includes the blue water footprint component (since impacts related to green and grey water resources would be assessed by other LCA indicators). Therefore, one approach is more appropriate for assessing water use in a water management context, while the other is more appropriate for evaluating various environmental impacts of products. Complementarities of both approaches are developed by Boulay et al. (2013).
Examples of studies that suggest that both water footprint methodologies are consistent:
– Bai, X., Ren, X., Khanna, N. Z., Zhang, G., Zhou, N., Bai, Y., & Hu, M. (2018). A comparative study of a full value-chain water footprint assessment using two international standards at a large-scale hog farm in China. Journal of Cleaner Production, 176, 557-565. https://www.sciencedirect.com/science/article/pii/S0959652617329220
– Jefferies, D., Muñoz, I., Hodges, J., King, V. J., Aldaya, M., Ercin, A. E., …Hoekstra, A. Y. (2012). Water Footprint and Life Cycle Assessment as approaches to assess potential impacts of products on water consumption. Key learning points from pilot studies on tea and margarine. Journal of Cleaner Production, 33, 155-166. https://www.sciencedirect.com/science/article/pii/S0959652612002028Hoekstra et al. (2011) acknowledge that uncertainty analyses are not available for water footprint accounting, not even approximate indications that show the degree of uncertainty in the various water footprint accounts. As for the LCA community, they recognize that frameworks to quantitatively account for uncertainty in a more rigorous manner are needed for water footprinting (Pfister et al., 2017).
For example, according to Hoekstra et al. (2011), in order to estimate the water footprint of growing crops, it is necessary to collect data such as crop parameters, crop yields, climate data, ambient water quality standards, and maps of soil, crops and irrigation. Since some data are not often available for various regions and obtaining location-specific data can be too difficult, assumptions are often made, and regional or national averages are used instead.
See Pimentel et al., (2004), and Chapagain & Hoekstra (2003).
They also calculated the water footprint of per protein and per fat, reaching the same conclusion: crop products are generally more efficient than animal products in delivering not only calories, but also protein, and fats (Mekonnen and Hoekstra, 2012).
The water footprint of concentrates in 5 times larger than roughages, according to Hoekstra (2012) and Gerbens-Leenes et al. (2013).
For example, the Netherlands presents lower water footprints for animal products than the U.S., and the U.S. presents lower water footprints for animal products than India. These crude general differences between countries are related to existing country differences in feed conversion efficiencies, but also to the fact that water footprints of feed crops vary across countries as a function of differences in climate and agricultural practice (Mekonnen & Hoekstra, 2010).
General Conclusions
The carbon and water footprints are quantitative methods to assess two types of environmental impacts, which implies they are not designed to be the sole basis for decision and policy making as a response to environmental challenges. However, they are still useful methods to get a general idea of the carbon and water use associated with products and diets.
Although the uncertainties involved in data and methodological choices are significant for estimating the carbon and water footprints of agricultural products, there are trends that are suggestive of what products and diets are more environmentally friendly than others, regarding climate change and water scarcity. Results of many studies suggest that carbon footprints of animal products are generally larger than carbon footprints of vegetal products, even without considering the emissions resulting from induced land change. Since the carbon footprint of diets depends on the carbon footprint of individual products, plant-based diets also tend to have smaller carbon footprints than animal-based diets. Even by constructing hypothetical scenarios, a plant-based diet seems to be the best type of diet to reduce dietary carbon footprints. Similar results in water footprint estimates suggest that, even without considering many forms of water pollution caused by animal production, animal products are generally more water-intensive than vegetal products. In the same line, studies that estimate the water footprint of dietary scenarios indicate that vegetarian diets have the highest potential to reduce dietary water footprints.
The main reason why this pattern is evidenced in the case of carbon and water footprinting, and perhaps on all environmental aspects considered altogether, is just that producing animals for food is inefficient from an environmental perspective. The impacts of animal products and animal diets always include the impacts of the agricultural crops and pastures produced to feed farmed animals, and on top of that the impacts caused by the animals directly, including pollution from manure and enteric fermentation. Therefore, from an environmental perspective, it’s always more efficient to eat vegetables directly, instead of giving them to the animals, and then eating the animals.
Further Considerations
Those environmentalists who take seriously the results from studies of the carbon and water footprint of products and diets, and promote plant-based diets as the best strategy to tackle dietary impacts related to climate change and water scarcity, might be potential allies of those animal advocates who recommend veganism as a strategy to help animals. Members from both movements could join efforts to promote plant-based diets as desirable from (at least) two different perspectives, perhaps reaching a more diverse audience, and creating opportunities for dialogue and solidarity between the two communities.
Although the ecological (or land) footprint was not addressed here, it is also important to note that the same pattern may also be expected. Animal products and animal-based diets not only release more GHG emissions, and use and pollute more water, but also seem to use and degrade more land than vegetable products and plant-based diets. Moreover, it would be worth examining all environmental impacts of diets in order to have a more comprehensive view on dietary impacts.
Research regarding the potential conflicts between environmentally friendly dietary recommendations and animal welfare dietary recommendations is needed, especially in cases where environmentalists focus on promoting the consumption of animal products that seem to be the most environmentally friendly rather than promoting plant-based diets. For example, it could be suggested that the consumption of animal products coming from systems with particular production techniques could be better for the environment than others, or that we should eat those types of animal products that have the smallest environmental footprints. Recommendations of this kind may support production systems that increase animal suffering, creating a conflict between the two communities. Something similar might happen in cases where animal advocates abstain from promoting plant-based diets and instead focus on promoting types of animal production systems that seem to be better in terms of animal welfare, but which may end up having larger environmental impacts. Identifying and examining these conflicts could create an opportunity to further the dialogue between environmentalists and animal advocates.
It would be worth comparing plant-based diets and animal diets in terms of other kinds of impacts, such as impacts on human health and food security, and even potential impacts on the welfare of wild animals.
Questions for further consideration
About the relations between environmental impacts and animal welfare:
- How the welfare of wild animals is affected by climate change, water pollution, and other types of environmental degradation?
- Is it possible that some wild animals could benefit from climate change, water pollution or other types of environmental degradation?
- Would it be plausible to prioritize environmental impacts in terms of their effects on animal welfare?
About footprints as indicators of environmental impacts:
- Are there more accurate indicators of environmental degradation caused by our dietary patterns?
- How accurate quantitative indicators such as LCAs are to assess environmental impacts of food products?
- Is there enough evidence to promote types of diets as environmentally friendly?
About estimates of dietary footprints:
- What is the contribution of food wastage in dietary footprints?
- What is the role of soil carbon sequestration, and direct and indirect land use change in carbon footprints of animal products and diets?
- How different water footprints of products would be if all sources of pollution were included?
About environmental impacts of agricultural systems:
- How effective are agricultural production techniques and technologies regarding environmental impacts?
- What kinds of food products have large water footprints but small carbon footprints, and the other way around?
- How environmental impacts of products differ across countries and regions?
About other types of impacts of food products and diets:
- What are the impacts of food products regarding biodiversity loss, land degradation, eutrophication, toxicity and other environmental issues?
- What kind of diets are the best not only in terms of environmental effects and animal welfare, but also regarding human health and food security?
Audsley, E., Brander, M., Chatterton, J., Murphy-Bokern, D., Webster, C., and Williams, A. (2009). How low can we go? An assessment of greenhouse gas emissions from the UK food system and the scope to reduce them by 2050. WWF-UK. Retrieved from https://www.fcrn.org.uk/fcrn/publications/how-low-can-we-go
Bai, X., Ren, X., Khanna, N. Z., Zhang, G., Zhou, N., Bai, Y., & Hu, M. (2018). A comparative study of a full value-chain water footprint assessment using two international standards at a large-scale hog farm in China. Journal of Cleaner Production, 176, 557–565. Retrieved from https://www.sciencedirect.com/science/article/pii/S095965261732922
Baroni, L., Cenci, L., Tettamanti, M., & Berati, M. (2007). Evaluating the environmental impact of various dietary patterns combined with different food production systems. European Journal of Clinical Nutrition, 61, 279–286. Retrieved from https://www.ncbi.nlm.nih.gov/pubmed/17035955
Becker, W., Welten, D. (2001). Under-reporting in dietary surveys – implications for development of food-based dietary guidelines. Public Health Nutrition, 4, 683–687. Retrieved from https://www.ncbi.nlm.nih.gov/pubmed/11683562
Berners-Lee, M., Hoolohan, C., Cammack, H., & Hewitt, C. (2012). The relative greenhouse gas impacts of realistic dietary choices. Energy Policy, 43, 184–190. Retrieved from https://www.sciencedirect.com/science/article/pii/S0301421511010603
Boulay, A., Hoekstra, A. Y., & Vionnet, S. (2013). Complementarities of Water-Focused Life Cycle Assessment and Water Footprint Assessment. Environmental Science & Technology, 47 (21), 11926–11927. dx.doi.org/10.1021/es403928f
British Standards Institution. (2011). PAS 2050:2011 Specification for the assessment of the life cycle greenhouse gas emissions of goods and services. United Kingdom: BSI. Retrieved from http://shop.bsigroup.com/upload/shop/download/pas/pas2050.pdf
Caffrey, K. C., & Veal, M. W. (2013). Conducting an Agricultural Life Cycle Assessment: Challenges and Perspectives. The Scientific World Journal. http://dx.doi.org/10.1155/2013/472431
Carlsson-Kanyama, A., & González, A. D. (2007). Non-CO2 greenhouse gas emissions associated with food production: methane (CH4) and nitrous oxide (N2O). KTH report Trita-IMA 2007: 22. Retrieved from: http://citeseerx.ist.psu.edu/viewdoc/download?doi=10.1.1.468.2795&rep=rep1&type=pdf
Chapagain & Hoekstra (2003). Virtual Water Flows between Nations in relation to Trade in Livestock and Livestock Products. Delft: UNESCO-IHE. Retrieved from http://www.ayhoekstra.nl/pubs/Report13.pdf
Clune, S., Crossin, E., Verghese, K. (2017). Systematic review of greenhouse gas emissions for different fresh food categories. Journal of Cleaner Production, 140, 766–783. Retrieved from https://www.sciencedirect.com/science/article/pii/S0959652616303584
de Vries, M., & de Boer, I. J. M. (2010). Comparing environmental impacts for livestock products: A review of life cycle assessments. Livestock Science, 128, 1–11. Retrieved from https://www.sciencedirect.com/science/article/pii/S1871141309003692
Extensive Agriculture. (n.d.). In Encyclopaedia Britannica online. Retrieved from https://www.britannica.com/topic/extensive-agriculture
Ercin, A. K., Aldaya, M. M., & Hoekstra, A. Y. (2012). The Water Footprint of Soy Milk and Soy Burger and Equivalent Animal Products. Ecological Indicators, 18, 392–402. Retrieved from http://waterfootprint.org/media/downloads/Ercin-et-al-2012-WaterFootprintSoy_1.pdf
FAOSTAT. (n.d). Food Balance Sheets. Retrieved from http://www.fao.org/faostat/en/#data/FBS
Food and Agriculture Organization. (n.d.). The use of concentrate feeds in livestock production systems. Retrieved from http://www.fao.org/wairdocs/lead/x6123e/x6123e04.htm
Garnett, T., Godde, C., Muller, A., Röös, E., Smith, P., de Boer, I. J. M… van Zanten, H. (2017). Grazed and confused? Ruminating on cattle, grazing systems, methane, nitrous oxide, the soil carbon sequestration question – and what it all means for greenhouse gas emissions. Food Climate Research Network. University of Oxford.
Garnett, T. (2008). Cooking up a storm: Food, greenhouse gas emissions and our changing climate. Food Climate Research Network. Retrieved from https://www.fcrn.org.uk/fcrn/publications/cooking-up-a-storm
Gerbens-Leenes, P. W., Mekonnen, M. M., & Hoekstra, A. Y. (2013). The Water Footprint of Poultry, Pork and Beef: A Comparative Study in Different Countries and Production Systems. Water Resources and Industry, 25–36. Retrieved from http://waterfootprint.org/media/downloads/Gerbens-et-al-2013-waterfootprint-poultry-pork-beef_1.pdf
Gerber, P. J., Steinfeld, H., Henderson, B., Mottet, A., Opio, C., Dijkman, J., Falcucci, A. & Tempio, G. (2013). Tackling climate change through livestock – A global assessment of emissions and mitigation opportunities. Rome: FAO. Retrieved from http://www.fao.org/3/a-i3437e.pdf
Greenhouse Gas Protocol. (2011). Product Life Cycle Accounting and Reporting Standard. USA: World Resources Institute and World Business Council for Sustainable Development. Retrieved from http://www.ghgprotocol.org/sites/default/files/ghgp/standards/Product-Life-Cycle-Accounting-Reporting-Standard_041613.pdf
González, A. D., Frostell, B., & Carlsson-Kanyama, A. (2011). Protein efficiency per unit energy and per unit greenhouse gas emissions: Potential contribution of diet choices to climate change mitigation. Food Policy, 36, 562–570. https://www.sciencedirect.com/science/article/pii/S030691921100090X
Hallström, E., Carlsson-Kanyama, A., & Börjesson, P. (2015). Environmental impact of dietary change: a systematic review. Journal of Cleaner Production, 91, 1–11. Retrieved from https://www.sciencedirect.com/science/article/pii/S0959652614012931
Hoekstra, A. Y. (2016). A critique on the water-scarcity weighted water footprint in LCA. Ecological Indicators, 66, 564–573. Retrieved from https://www.sciencedirect.com/science/article/pii/S1470160X16300474
Hoekstra, A. Y. (2012). The Hidden Water Resource Use Behind Meat and Dairy. Animal Frontiers, 2 (2), 3–8. Retrieved from http://waterfootprint.org/media/downloads/Hoekstra-2012-Water-Meat-Dairy.pdf
Hoekstra, A. Y., Chapagain, A. K., Aldaya, M. M., & Mekonnen, M. M. (2011). The Water Footprint Assessment Manual: Setting the Global Standard. London: Earthscan. Retrieved from http://waterfootprint.org/media/downloads/TheWaterFootprintAssessmentManual_2.pdf
Hoekstra, A. Y., & Hung, P. Q. (2002). Virtual water trade: a quantification of virtual water flows between nations in relation to international crop trade. The Netherlands: IHE DELFT. Retrieved from http://waterfootprint.org/media/downloads/Report11.pdf
Hoekstra, A. Y., Gerbens-Leenes, W., & Van der Meer, T. H. (2009). Reply to Pfister and Hellweg: water footprint accounting, impact assessment, and life-cycle assessment. Proceedings of the National Academy of Sciences of the United States of America, 106 (40), E114. Retrieved from http://www.pnas.org/content/pnas/106/40/E114.full.pdf
Hoekstra, A. Y., & Mekonnen, M. M. (2012). Reply to Ridoutt and Huang: from water footprint assessment to policy. Proceedings of the National Academy of Sciences of the United States of America, 109 (22), E1425. Retrieved from http://www.pnas.org/content/pnas/109/22/E1425.full.pdf
International Organization for Standardization. (2014). Environmental management — Water footprint — Principles, requirements and guidelines. (ISO Standard No. 14046). https://www.iso.org/standard/43263.html
International Organization for Standardization. (2006). Environmental management — Life cycle assessment — Principles and framework (ISO Standard No. 14040). Retrieved from https://www.iso.org/standard/37456.html
International Organization for Standardization. (2006). Environmental management — Life cycle assessment — Requirements and guidelines (ISO Standard No. 14044). Retrieved from https://www.iso.org/standard/38498.html
International Organization for Standardization. (2013). Greenhouse gases — Carbon footprint of products — Requirements and guidelines for quantification and communication (ISO/TS Standard No. 14067). Retrieved from https://www.iso.org/standard/59521.html
IPCC. (2014). Climate Change 2014: Synthesis Report. Contribution of Working Groups I, II and III to the Fifth Assessment Report of the Intergovernmental Panel on Climate Change [Core Writing Team, R. K. Pachauri and L. A. Meyer (eds.)]. Geneva, Switzerland: IPCC. Retrieved from http://www.ipcc.ch/report/ar5/syr/
Islam, M. S., Oki, T., Kanae, S., Hanasaki, N., Agata, Y., & Yoshimura, K. (2007). A grid-based assessment of global water scarcity. Water Resour Manage, 21, 19–33. Retrieved from https://link.springer.com/article/10.1007/s11269-006-9038-y
Jalava et al. (2014). Diet change—a solution to reduce water use? Environmental Research Letters, 9. doi:10.1088/1748-9326/9/7/074016
Jefferies, D., Muñoz, I., Hodges, J., King, V. J., Aldaya, M., Ercin, A. E, …Hoekstra, A. Y. (2012). Water Footprint and Life Cycle Assessment as approaches to assess potential impacts of products on water consumption. Key learning points from pilot studies on tea and margarine. Journal of Cleaner Production, 33, 155–166. Retrieved from https://www.sciencedirect.com/science/article/pii/S0959652612002028
Kummu, M., Ward, P. J., de Moel, H., & Varis, O. (2010). Is Physical Water Scarcity a New Phenomenon? Global Assessment of Water Shortage over the Last Two Millennia. Environ. Res. Lett., 5. Retrieved from http://iopscience.iop.org/article/10.1088/1748-9326/5/3/034006/meta
Mekonnen, M., & Hoekstra, A. Y. (2016). Four billion people facing severe water scarcity. Science Advances, 2 (2). Retrieved from http://advances.sciencemag.org/content/2/2/e1500323
Mekonnen, M. M., & Hoekstra, A. Y. (2012). A Global Assessment of the Water Footprint of Farm Animal Products. Ecosystems, 15, 401–415. Retrieved from https://link.springer.com/article/10.1007/s10021-011-9517-8
Mekonnen, M. M., & Hoekstra, A. Y. (2011). The green, blue and grey water footprint of crops and derived crop products. Hydrology and Earth System Sciences, 15, 1577–1600. Retrieved from http://waterfootprint.org/media/downloads/Mekonnen-Hoekstra-2011-WaterFootprintCrops.pdf
Mekonnen, M. M., & Hoekstra, A. Y. (2010). The Green, Blue and Grey Water Footprint of Farm Animals and Animal Products. Delft: UNESCO-IHE. Retrieved from http://waterfootprint.org/media/downloads/Report-48-WaterFootprint-AnimalProducts-Vol1.pdf
Neill, C., Steudler, P., Garcia-Montiel, D., Melillo, J., Feigl, B., Piccolo, M., & Cerri, C. (2005). Rates and controls of nitrous oxide and nitric oxide emissions following conversion of forest to pasture in Rondônia. Nutrient Cycling in Agroecosystems, 71, 1–15. Retrieved from https://link.springer.com/article/10.1007/s10705-004-0378-9
Nijdam, D., Rood, T., & Westhoek, H. (2012). The price of protein: Review of land use and carbon footprints from life cycle assessments of animal food products and their substitutes. Food Policy, 37, 760–770. Retrieved from https://www.sciencedirect.com/science/article/pii/S0306919212000942
Pelletier, N. (2007). Environmental performance in the US broiler poultry sector: Life cycle energy use and greenhouse gas, ozone depleting, acidifying and eutrophying emissions. Agricultural Systems, 98, 67–73. Retrieved from https://www.sciencedirect.com/science/article/pii/S0308521X08000401
Pfister, S., Boulay, A., Hadjikakou, M., Motoshita, M., Hess, T., Ridoutt, B., … Buxmann, K. (2017). Understanding the LCA and ISO water footprint: A response to Hoekstra (2016) “A critique on the water-scarcity weighted water footprint in LCA”. Ecological Indicators, 72, 352–359. Retrieved from https://www.sciencedirect.com/science/article/pii/S1470160X16304599
Pfister, S., & Hellweg, S. (2009). The water “shoesize” vs. footprint of bioenergy. Proceedings of the National Academy of Sciences of the United States of America, 106 (35), E93–E94. Retrieved from http://www.pnas.org/content/pnas/106/35/E93.full.pdf
Pfister, S., & Ridoutt, B. G. (2014). Water footprint: pitfalls on common ground. Environmental Science & Technology, 48 (1), 4. Retrieved from https://pubs.acs.org/doi/10.1021/es405340a
Pimentel, D., Berger, B., Filiberto, D., Newton, M., Wolfe, B., Karabinakis, E., … Nandagopal, S. (2004). Water Resources: Agricultural and Environmental Issues. Bioscience, 54 (10), 909–918. Retrieved from https://academic.oup.com/bioscience/article/54/10/909/230205
Ridoutt, B. G., & Huang, J. (2012). Environmental relevance – the key to understanding water footprints. Proceedings of the National Academy of Sciences of the United States of America, 109 (22), E1424. Retrieved from https://www.ncbi.nlm.nih.gov/pmc/articles/PMC3365216/pdf/pnas.201203809.pdf
Roy, P., Nei, D., Orisaka, T., Xu, Q., Okadome, H., Nakamura, N., & Shiina, T. (2009). A review of life cycle assessments (LCA) on some food products. Journal of Food Engineering, 90, 1–10. https://www.sciencedirect.com/science/article/pii/S0260877408002793
Röös, E., Tidåker, P., Strid, I., & Hansson, P. (2013). Can carbon footprint serve as an indicator of the environmental impact of meat production? Ecological Indicators, 24, 573–581. Retrieved from https://www.sciencedirect.com/science/article/pii/S1470160X12002956
Scarborough, P., Appleby, P. N., Mizdrak, M., Briggs, A. D. M., Travis, R. C., Bradbury, K. E., & Key, T. J. (2014). Dietary greenhouse gas emissions of meat-eaters, fish-eaters, vegetarians and vegans in the UK. Climatic Change, 125, 179–192. Retrieved from https://link.springer.com/article/10.1007/s10584-014-1169-1
Steinfeld, H., Gerber, P., Wassenaar, T., Castel, V., Rosales, M., & de Haan, C. (2006). Livestock’s Long Shadow: Environmental Issues and Options. Rome: FAO.
Swain, M., Blomqvist, L., McMara, J., & Ripple, W. J. (2018). The environmental impact of global diets. Science of the Total Environment, 610-611, 1207–1209. Retrieved from http://trophiccascades.forestry.oregonstate.edu/sites/trophic/files/Swain2017.pdf
Vanham, D., del Pozo, S., Pekcan, A. G., Keinan-Boker, L., Trichopoulou, A., Gawlik, B. M. (2016). Water consumption related to different diets in Mediterranean cities. Science of The Total Environment, 573, 96–105. Retrieved from https://www.sciencedirect.com/science/article/pii/S004896971631806X
Vanham, D., Hoekstra, A. Y., & Bidoglio, G. (2013). Potential Water Saving through Changes in European Diets. Environment International, 61, 45–56. Retrieved from http://waterfootprint.org/media/downloads/Vanham-et-al-2013_1_1.pdf
Water Footprint Network. (n.d.). Glossary. Retrieved from http://waterfootprint.org/en/water-footprint/glossary/
Werner, L. B., Flysjö, A., & Tholstrup, T. (2014). Greenhouse gas emissions of realistic dietary choices in Denmark: The carbon footprint and nutritional value of dairy products. Food & Nutrition Research, 58. http://dx.doi.org/10.3402/fnr.v58.20687
Williams, A. G., Audsley, E., & Sandars, D. L. (2006). Determining the environmental burdens and resource use in the production of agricultural and horticultural commodities. Main Report. Defra Research Project IS0205. Bedford: Cranfield University and Defra. Retrieved from http://randd.defra.gov.uk/Default.aspx?Module=More&Location=None&ProjectID=11442
Yan, M., Humphreys, J., & Holden, N. (2011). An evaluation of life cycle assessment of European milk production. Journal of Environmental Management, 92, 372–379. Retrieved from https://www.ncbi.nlm.nih.gov/pubmed/21055870